POWERING AUTONOMONY
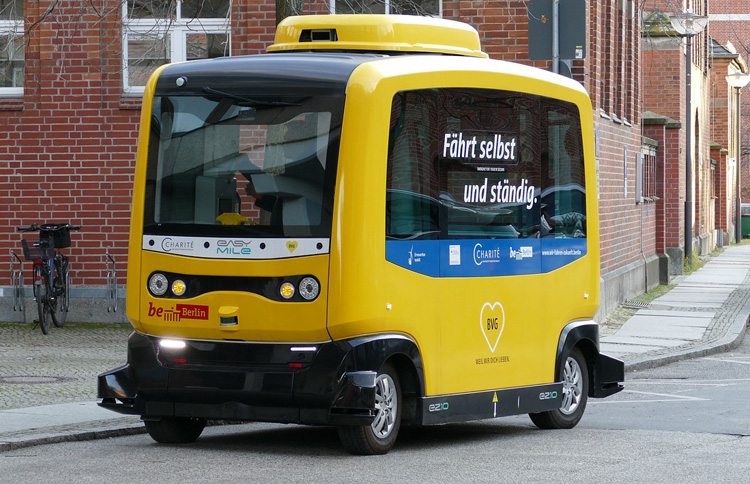
ENERGY-EFFICIENT BRAKING CRUCIAL TO SUCCESS OF AUTONOMOUS VEHICLES
When a self-driving Uber crash led to a fatality in 2018, to many, it seemed that the autonomous vehicle revolution was over before it had chance to gather speed. If autonomous vehicles are to make it onto our driveways, braking systems will be crucial to minimising accidents.
One year after the Uber crash, the National Transportation Safety Board (NTSB), an independent US government investigative agency, concluded that a major factor in the collision was a misjudgement by the vehicle’s safety operator — or, the human that sits in the vehicle and monitors the autonomous driving system.
While it seems that human error will never go away, manufacturers have not given up on the concept that the right technologies can help autonomous vehicles steer away from disaster. Automotive giants, like Jaguar Land Rover (JLR), continue to invest in autonomous technology. JLR’s Project Vector concept aims to have an “autonomy-ready” and “multi-use electric vehicle” on the road by 2021.
While it’s clear that the future will be self-driving, how will manufacturers avoid the accidents of the past — and what role can advanced braking systems play?
FOLLOW THE ROUTE
Operating from March 2018 to June 2019, the Route 12 driverless bus concept successfully provided Schaffhausen, Switzerland with a driverless bus system over a year. Big cities can learn a lot from the small Swiss town and, last year in Germany, Berlin’s public transport company, Berliner Verkehrsbetriebe (BVG), also tested out its own autonomous buses.
Autonomous transport offers many benefits to towns and cities alike, not least in terms of safety. The UK’s Department for Transport reports that 27,820 people were killed or seriously injured in reported road traffic accidents in the year ending June 2019. Leading causes for these accidents included speeding, lack of focus or driving under the influence — all of which are results of human error.
Programmable driving systems should eliminate these unsafe human habits, of course. In addition, driverless vehicles can also reduce traffic congestion by following fixed routes that are simpler to handle than the various and complicated routes along which a taxi or car usually travels.
REGENERATIVE BRAKING
Choosing the most effective system for an autonomous vehicle goes beyond merely bringing the vehicle to a stop. As most autonomous vehicles in the future are expected to be electric vehicles (EVs), braking systems will also play a crucial role in optimising energy consumption.
This applies to EVs used for public transport, where multiple stops and starts along a single route are an energy drain. When these stops occur, and because the electric motor behaves like a generator under these conditions, the EV releases energy that is fed back into the drive system. This energy needs somewhere to go, so heads towards the EV’s battery as part of a process is known as regenerative braking.
If the battery is full, and the EV has no other means to dissipate the excess energy, then the speed of the vehicle might be limited in order that the mechanical brakes can safely bring the vehicle to a stop without the possibility of causing brake fade or failure.
To remedy this, a braking resistor, such as Cressall’s EV2, can dissipate excess energy when the battery does not accept the charge. This type of braking is known as dynamic braking. Wherever possible, braking should be regenerative rather than mechanical. This creates the possibility of storing and re-using braking energy, rather than just dissipating it as waste heat.
Furthermore, many public service and heavy goods vehicles are fitted with auxiliary or endurance braking systems that work in tandem with the service brakes. The EV2 is an ideal substitute to these mechanical, hydraulic or magnetic systems.
Heating also plays an important role in making use of this regenerated energy. The EV2 is a liquid cooled resistor. Specifically, it is cooled by pumping the cold liquid that comes into one end of the system, which then absorbs the heat generated by the resistor. This heated liquid can be pumped through a radiator, then used to provide heat to the cabin of the vehicle for a more comfortable passenger experience.
This method of heating reduces the amount of energy required from the battery, and uses heat that would otherwise have been wasted.
While the wide-spread adoption of autonomous transport has yet to become a reality, it’s not difficult to imagine the day when cars will brake on their own, as commanded by autopilot. After all, electric steering systems already perform a similar function. Braking technology can’t change the mistakes of the past, but it can be a huge driver in delivering energy-efficient autonomous transport.
To find out more about Cressall’s EV2 for electric vehicles, click here